Program Structure
-
Home Item
-
General Item
-
General Item
-
General Item
The Need
As industry leaders and professional organizations consider the future of their work in the twenty-first century, they believe that the major innovations and discoveries will be found at the cross-section of different areas of expertise. They expect that knowledge, types of expertise, and structures of employment will continuously evolve, requiring life-long learning skills and acceptance of ambiguity and continuous change.
The Calhoun Discovery Program aims to provide a foundation for Virginia Tech students from multiple disciplines to move beyond disciplinary boundaries to solve complex problems, identify new problems, and work as collaborative lifelong learners, preparing them to be adaptive global citizens.
The Integrative Curriculum Model
For the first phase of the program, we have selected the theme of Collaborative Technology Innovation for Societal Impact (with a special focus on computing, information, and decision technologies). This theme combines Virginia Tech’s strength in technology across multiple disciplines with our land-grant mission for societal impact. Our knowledge integration approach builds on established models for developing highly-engineered systems within complex societal contexts and for advancing multidisciplinary technology innovation [1,2,3,4,5,6].
The images below summarize the structure of our integrative curriculum. The external quadrants denote the knowledge components necessary for integrative technology innovation: feasibility, viability, desirability, and sustainability. These knowledge components will be covered by twelve participating disciplines and units at Virginia Tech. Their placement on the quadrants shows their approximate knowledge coverage. The two middle circles show a two-stage integration process:
- Development of collaboration and communication skills across the different areas of knowledge through interdisciplinary general education
- Knowledge integration through applied transdisciplinary projects.
The three circles have a bidirectional relationship: integration experiences inform the understanding of the components, and vice versa.
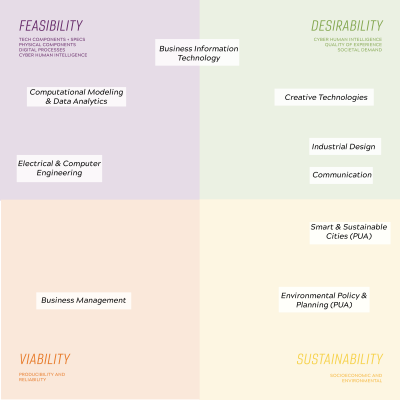
Stage 1
The four quadrants denote the knowledge components necessary for integrative technology innovation, covered by twelve participating disciplines and units at Virginia Tech. Their placement on the quadrants shows their approximate knowledge coverage.
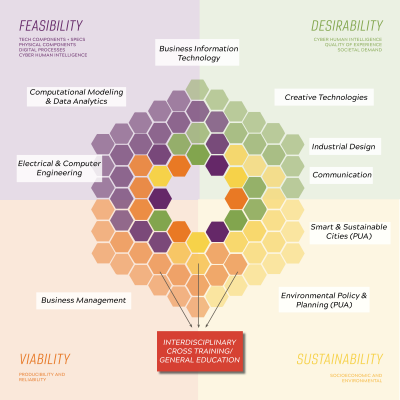
Stage 2
Interdisciplinary general education: students learn about the skills of their collaborators and develop crosscutting skills.
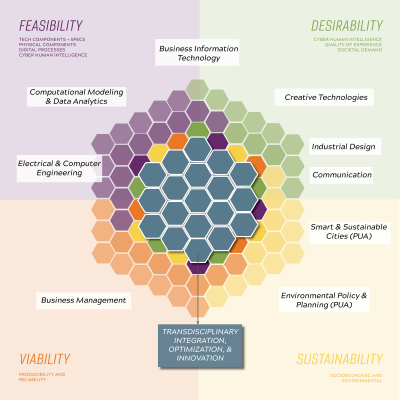
Stage 3
Knowledge integration through applied transdisciplinary projects.
Interdisciplinary General Education
A significant part of the general education experience of the Discovery Program focuses on cross-training that facilitates diverse collaborations. A student will learn to connect key threshold concepts from their discipline with relevant concepts from the disciplines of other students with whom they will collaborate. Each threshold concept will be taught through a one-credit module. Each module introduces the concept and its sub-concepts as well as connects the concept to the technology innovation integration model of the Discovery Program. All modules employ a flipped-classroom format, combining rich faculty-student interactions online and in person, which facilitates adaptive learning and flexible scheduling. Their placement in the categories below shows the initial anchor quadrant of each module. However, modules will have connections to all four quadrants. Students can combine modules across quadrants to fullfil pathways general education requirements. As the program evolves, the number of available modules will increase and the content will change.
- Algorithmic thinking
- Computing systems
- Acquiring & analyzing data
- Estimation, expectation, descriptive analytics
- Prescriptive & predictive analytics
- Matrix Computation and Calculation
- Artificial Intelligence & Machine Learning
- Decisions & Simulations
- Dynamic Systems
- Hardware / Software Interface
- Signal Processing
- Transducers & Circuits
- Power & Energy
- Optimization & Robustness
- Fundamentals of manufacturing processes
- Security and Privacy
- Operations Management
- Entrepreneurship
- Market Analysis
- Iteration and [Continuous] Improvement
- Supply Chain Management
- Aesthetics
- Interaction
- Form
- Contexts for Technology Design
- Communicating Data
- Professional Media Writing
- Speech and Professional presentation
- Visual Media Communication
- Multimedia & Broadcast Communication
- Online Collaboration tools
- Human Environment Interaction
- Human Condition and Structural Inequity
- World Economy
- Technology Systems + Economic Development
- Social Construction of Theory
- Systems & Systems Thinking
- Community & Identity
- Digital Media & Society
Transdisciplinary studios
Faculty and students from all participating disciplines, as well as experts from industry and non-profit partners, will come together for hands-on explorations of real-world problems. The problem spaces that they tackle will span strategic areas of discovery for our partners, students, and faculty. The Discovery collaboration and prototyping lab is available to students, faculty, and partners 24/7 for collaborative exploration of these problems spaces. The exploration is also facilitated by a virtual platform for distributed collaboration.
Students take one semester-long collaborative studio in their first two years and one studio per semester in the third and fourth year of studies. The Discovery studios count towards disciplinary experiential learning requirements, including the senior capstone. Capstone studios may be partly realized in partner facilities and locations. Summer and wintermester internships with partners may also be connected to the studios.
The focal areas of each studio are as follows:
First-year studio (Fall):
Collaborative problem-setting; Global contexts for technology innovation spanning feasibility, viability, desirability, sustainability; Reflective making; Ethical dimensions of collaborative technology innovation for societal impact.
Second-year studio (Spring):
Collaborative problem-solving; Introduction to quantitative and qualitative research methods; Optimization and integration; Component prototyping; Ethical dimensions of collaborative technology innovation for societal impact
Third-year studios:
(Fall): Systems thinking and systems definition; Identification and analysis of stakeholder; Skills discovery and transdisciplinary team building; Rapid Prototyping.
(Spring): Collaborative innovation; Customer discovery; Evidence-based decision-making; Iterative Design; Troubleshooting.
Fourth-year (Capstone) studios:
(Fall): Systems building; Project leadership and management, including resource allocation and scheduling; Team management; Value propositions. Project pitching
(Spring): User experience; User Testing; Systems assessment addressing feasibility, viability, desirability, sustainability, optimization, and integration. Systems reflection and documentation
The discovery and knowledge integration process
As the students improve in their collaborative projects and discover their interests and strengths, they can select which area they want to focus on within their discipline and what concepts from other disciplines they want to learn. Everything the students learn in their discipline is continuously recontextualized by being connected to relevant concepts from other disciplines and to complex real-world projects. The students can potentially develop knowledge identities that merge two or more disciplines. This merging does not take the form of a list of multiple majors or minors. Instead, it is a set of embodied and socially embedded skills that the student can intuitively combine and apply to the complexity of their lived experience and to advancing comprehensive approaches to societal challenges.
In a traditional interdisciplinary collaboration model, each student is assumed to have a fixed base within their discipline and to be connected to experts from other disciplines through collaborative projects. In the Discovery model, the student is a life-long transdisciplinary learner who is able to recruit an evolving array of disciplinary toolsets across multiple disciplines as well as diverse collaborators to facilitate a meaningful exploration of life and society.
In the traditional disciplinary education model, the student takes their fixed-knowledge identity from their discipline: “I am an engineer” or “I am an artist,” for example.
In the Discovery model, the student takes their evolving knowledge identity from their explorations: “I am exploring inequality in education by combining my knowledge of engineering and arts through collaboration with social scientists, members of underserved communities, and business that are interested in serving these communities”.
Or “I am currently exploring semi-automated air delivery for goods as a means of decreasing congestion and pollution in cities by combining my knowledge in business, design, and big data and collaborating with engineers and policymakers”. Thus, in our visual representation of the Discovery program structure, each student becomes a star with a transdisciplinary core and a unique and ever-evolving collection of disciplinary rays, with one of those rays being their disciplinary anchor.
As participants’ knowledge evolves, the initial quadrants of knowledge in the Discovery model gradually shift and merge. For example, a great technological insight can come from a Discovery program student anchored in industrial design and a new policy perspective from a Discovery program student anchored in engineering. Through these collaborations, the students learn to look to the uniqueness of their colleagues, rather than to disciplinary tags, for structuring their collaborations.
References
- Miller, R. From the Ground Up: Rethinking Engineering Education for the 21st Century. In Symposium on Liberal Education, Union College, New York, 2010: Union College
- Olivier L. de Weck A, Daniel Roos A, Christopher L. Magee A, Charles M. Vest A. Engineering Systems : Meeting Human Needs in a Complex Technological World. MIT Press; 2011.
- Engines, GE Aircraft et al. “1 FIPER : An Intelligent System for the Optimal Design of Highly Engineered Products.” (2005)
- Page, S. E. (2007). The difference. Princeton: Princeton University Press.
- Herbert A. Simon A. The Sciences of the Artificial. MIT Press; 1996.
- Nicolescu, B. (2002). Manifesto of transdisciplinarity (SUNY series in Western esoteric traditions). Albany: State University of New York Press.